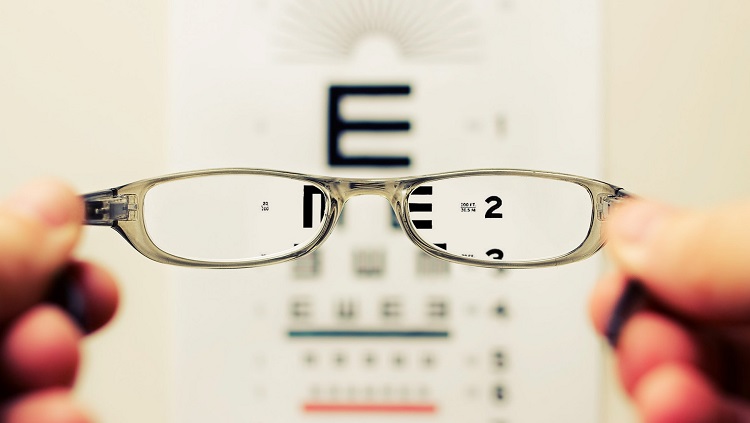
In 1992, Benjamin Spencer’s vision went black. Specialists diagnosed him with pediatric glaucoma, a condition where fluid builds up inside the eye and doesn’t drain properly. The pressure eventually damages the optic nerve and causes vision.
Twenty-seven years later, Spencer participated in a study to restore his sight. Wearing glasses with cameras wired into his brain, Spencer sees light framed by doorways and windows. The device works because most aspects of vision occur in the brain.
Vision is complicated — many cells and molecules work together to help you see. First, light slips through the cornea and enters through the pupil. The lens bends light, focusing it onto the retina, a sheet of cells lining the inner surface of your eyeball. Like a camera capturing images, light on the retina creates a two-dimensional reversed image. Objects to the right project images on the left and vice versa. Objects above are imaged on the lower part and vice versa.
The Three-Layered Retina
The retina houses photoreceptors, interneurons, and ganglion cells. Neurons communicate with each other before sending information to the brain. First, photoreceptors — rods and cones — respond to light. Located in the retina’s outer layer, light passes through ganglion cells and interneurons before reaching rods and cones. Photoreceptors send signals to ganglion cells and interneurons, which process and relay information out of the retina.
Photoreceptors turn light into electrical signals. Extremely sensitive, rods let you see in dim light. Cones are responsible for color and detail. Your eye has three types of cones, each sensitive to a different range of colors: red, green, or blue. We see more colors because each cone reacts to every color.
The center has more cones than any other area, so vision is sharper here than in the periphery. In the very center lies the fovea, where cones are most densely packed. It contains red and green cones and resolves details. The fovea and the area around it, the macula, are responsible for high-resolution vision for reading and driving.
How many cells each neuron talks to varies across the retina. In the macular region, each ganglion cell receives input from very few cones, letting you see details. Near the edges, each cell gets signals from several photoreceptors, so peripheral vision is less detailed.
Processing Visual Information
Imagine you’re in a forest. The tree canopy creates patchworks of sunlight and shadow across the understory and forest floor. The eye captures and projects light onto the retina. How does the brain process a two-dimensional retinal image to create a complex scene?
First, the brain compares amounts of light hitting small, adjacent areas on the retina. The visual space providing input to a ganglion cell is its receptive field. Receptive fields “tile” the retina, providing a two-dimensional map of the scene. A receptive field activates when light hits the region on the retina corresponding to the center of its field. It’s inhibited when light hits the donut-shaped area surrounding the center. If light strikes the entire field — the donut and its hole — the ganglion cell responds weakly. How these cells respond to light is one way our vision perceives contrast, which helps us detect objects.
The forest’s light and dark patterns activate ganglion cells in a manner corresponding to the surrounding scene. Cells send signals along optic nerves to the brain. There are no photoreceptors where the optic nerve meets the retina, creating a “blind spot.” Our brains fill the gap using information from the other eye. Signals traveling along nerve fibers from both eyes meet at the optic chiasm. Fibers carrying information from both retinas’ left sides continue together on the brain’s left side; information from the right goes to the brain’s right. Visual information moves through the thalamus, then goes to the primary visual cortex.
The Visual Cortex
Located in the occipital lobe, the primary visual cortex has layers with densely packed cells. The middle layer receives messages from the thalamus, has receptive fields, and preserves the retina’s visual map. Cells above and below have more complex receptive fields, registering bar shapes, edges, or stimuli with particular orientations. Specific cells respond to an object’s edges at certain angles. Other cells respond to objects moving in a direction, like birds flying from left to right. From these layers, new processing streams pass information to other parts of the cortex. Receptive fields in these parts become more complex and selective as they combine visual information.
Visual signals go into parallel but interacting processing streams. The dorsal stream heads to the parietal lobe, which combines sensory information. The ventral stream goes to the temporal lobe, which governs decision-making and conscious thought. Traditionally, researchers thought these streams processed unconscious vision, which guides behavior, and conscious vision separately. Seeing a dog run into the street, the ventral stream integrates information about its shape and color with past experiences to recognize the dog is your neighbor’s. The dorsal stream combines spatial relationships, motion, and timing to create an action plan without conscious thought. You may shout “stop!” without thinking. Ongoing research questions this strict division, suggesting crosstalk between streams may create a conscious experience.
Eyes Come in Pairs
Each eye sees an object from a different angle, letting you perceive depth, or three dimensions. It works if both eyes are equally active, properly aligned, and their visual fields overlap. Because some nerve fibers exiting each eye cross over at the optic chiasm, signals from the left visual field go to the brain’s right and vice versa. Each half of the cerebrum processes information from the opposite side of the body.
Visual Disorders
People with strabismus — crossed eyes — don’t have much depth perception. Initially, children have good vision in each eye. Since they can’t fuse images coming from both eyes, they favor one and lose vision in the other. If children receive treatment while they’re young, vision can be restored. Around age eight, blindness becomes permanent. Until recently, doctors waited until age four before operating to align children’s eyes and instead prescribed exercises or using an eye patch. With improved techniques, doctors now perform surgery even earlier to correct strabismus.
Many disorders causing blindness occur when photoreceptors die or stop functioning. Researchers are identifying genetic defects that cause blindness, which could lead to therapies that recover rods and cones. Gene therapies already help some patients with macular degeneration or other forms of blindness see better.
Scientists are examining ways to send electrical signals to the brain via ganglion cells. The work includes research that helped Spencer, but it won’t be able help people born blind – their brains never learned how to process visual information. Researchers continue studying vision to find even more effective treatments.
Adapted from the 8th edition of Brain Facts by Sandra Blumenrath.
CONTENT PROVIDED BY
BrainFacts/SfN
References
Childhood Glaucoma | Glaucoma Research Foundation. (n.d.). Retrieved December 13, 2019, from https://www.glaucoma.org/glaucoma/childhood-glaucoma-1.php
Gutierrez, G. (2019, July 11). Orion shines a light in the dark for the blind. Retrieved December 13, 2019, from Baylor College of Medicine Blog Network website: https://blogs.bcm.edu/2019/07/11/from-the-labs-orion-turns-on-a-light-in-the-dark-for-the-blind/