Teaching the Brain to See Again
- Published24 May 2018
- Reviewed24 May 2018
- Author Rachel Kaufman
- Source BrainFacts/SfN
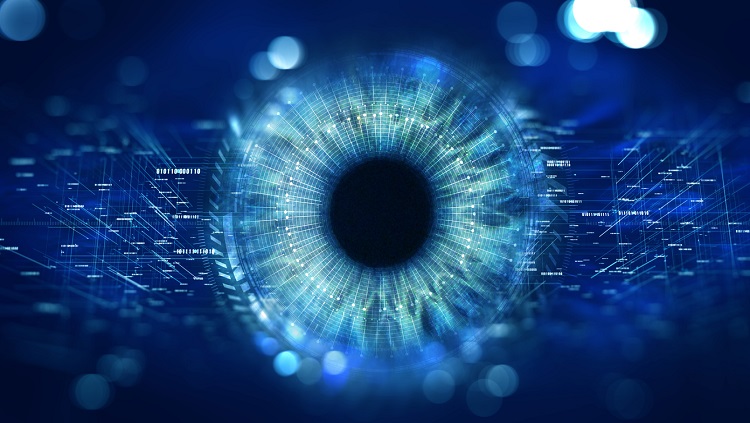
What if you could flip a switch and restore vision to a blind person? It sounds near-miraculous. And, yet, since the 1970s scientists have been trying to do just that. If they succeed, it won’t be a miracle. It will be a triumph of neuroscience and technology.
William Dobelle created the first “bionic eye” technology roughly four decades ago. Comprised of a tiny camera mounted in eyeglasses, a portable computer, and electrodes implanted in brain, the “Dobelle Eye” in effect used technology to mimic the electrical stimulation from the retina and optic nerve that results in vision.
The Dobelle Eye was first implanted in a blind person in 1978. However, the system wasn’t turned on and tested out until 2000. And, it worked, briefly. The system ultimately failed, and nobody knows why. Dobelle died in 2004 before he could come up with a solution.
Now, researchers around the world are employing advanced microarrays, computers and cameras to develop visual prostheses based on the same principles that informed Dobelle’s original technology.
When you rub your eyes too hard or are unfortunate enough to experience a blow to the head, you often see “spots” or “stars” — please don’t try this at home. Scientists call those perceived flashes of lights phosphenes, and they underpin the efforts to use electricity to stimulate the visual cortex, the part of the brain that processes sensory information from the eyes. By stimulating it in the right way, researchers can trigger the production of phosphenes. And, your brain “thinks” it is seeing light, even though it is not. For years, researchers have stimulated the brain with electrical pulses creating these phosphenes; now, scientists like Philip Troyk and Ken Shepard are trying to use them to teach the brain to see.
Early versions of “bionic eyes,” including Dobelle’s, had very low resolution, meaning that they could create only a few phosphenes at a time, leading to a blurry, or pixelated, view of the world.
Troyk, who is Associate Dean of Armour College of Engineering at the Illinois Institute of Technology, is leading a team aiming to build a visual prosthesis, supported by a five-year $11.8 million grant from the NIH BRAIN Initiative. Troyk’s team is attempting to build a device with implantable electrodes that can stimulate up to 1000 sites in the visual cortex. This is still, he says, a “crude” imitation of vision, since the human retina has 10 million receptors.
As such, the device, currently known as the IntraCortical Visual Prosthesis Project or ICVP, will certainly not “make the blind see again.” But assuming the device works, Troyk says, it “could be very useful to people who are without vision.”
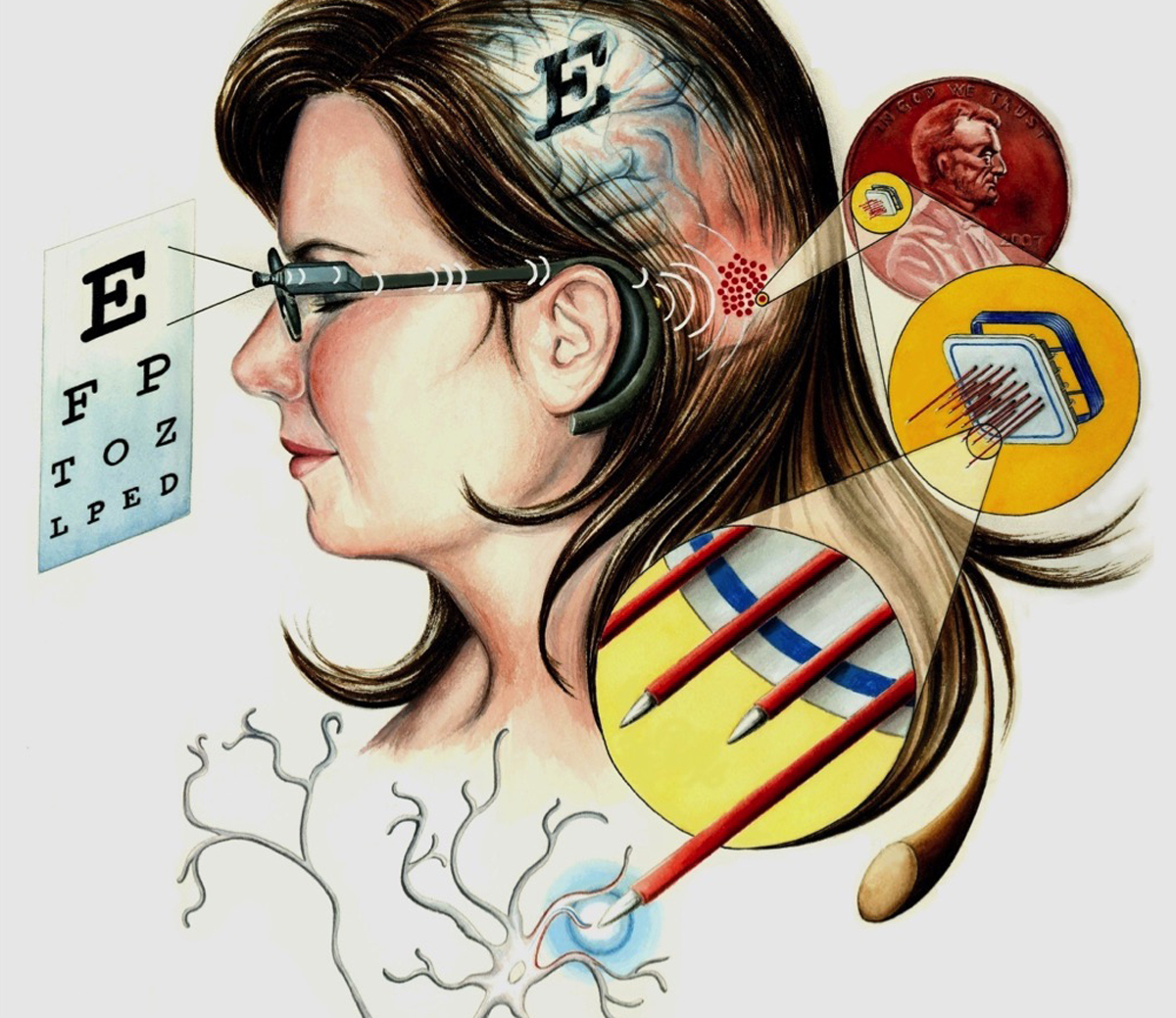
Both Troyk and Shepard are building devices that use the same basic principle of the Dobelle Eye — electrical stimulation of the visual cortex — but they are significantly more advanced.
While Troyk’s device, when completed, will be implanted in the brain, Shepard’s vision involves employing a thin electrode array that rests on the surface of the brain. Shepard, professor of electrical engineering and biomedical engineering at Columbia University, and a team of scientists are developing an implantable electrode array only 15 microns thick, about the same as three human red blood cells next to each other.
“At those thicknesses, an integrated circuit is like a piece of paper,” Shepard says. “You can bend it and drape it over the surface of the brain.” Shepard’s device, when completed, will be inserted in a “relatively” noninvasive surgery and rest on the surface of the visual cortex. His team is planning to create a device with one million electrodes to stimulate individual neurons. Shepard is also supported by the U.S. BRAIN Initiative, under DARPA’s Neural Engineering System Design program and has also received funding from the NIH for other work on arrays.
Both Troyk’s and Shepard’s devices will connect the electrodes to a camera that can convert what the user is seeing into data. Computer algorithms will determine the best way to translate the visual data, sending signals to specific parts of the brain, which will stimulate the production of phosphenes that the user can "see." But, users won’t immediately get “vision.” The user would need time to get used to how to interpret the signals from their brain as visual information.
“There would have to be a period of adaptation and training, just like any new skill,” Troyk says.
“What you're relying on is the plasticity of the brain,” says Shepard. “The human brain is adaptable.” This means that the brain can learn to interpret signals as visual information, even if they’re not the same signals the person is used to receiving through their optic nerve. Those signals can be electrical pulses, as in Troyk’s and Shepard’s devices, or they can be information from other parts of the brain.
For example, some studies have looked at the few blind people who have taught themselves to navigate the world through echolocation, or the use of reflected sound as a navigation aid. One of the most well-known blind "echolocators" is Daniel Kish, an advocate for the blind, who teaches others how to use sound to navigate. A study using fMRI to look at the brains of both echolocators and control subjects found that when echolocators heard echoes, their visual cortices were active. Blind people who read Braille show activation in their visual cortices, but sighted people performing the same task don’t. Are these activities, then, “sight?” Scientists aren't sure.
Both Troyk and Shepard believe that it is likely that their devices will only work for people who had sight in the past but lost it due to trauma.
“The prevailing hypothesis is you need to have developed the neural machinery of vision before one could tap into that machinery and manipulate it,” says Troyk. But then, he adds, “We’re not sure.”
Cochlear implants, devices for people who are deaf or hard of hearing that work by directly stimulating the auditory nerve, have been implanted in people at younger and younger ages with good results. Troyk believes that similarly, if a baby without sight had a visual prosthetic implanted very early, “then the plasticity of that system could then adapt.” It’s early yet and that hypothesis is, for now, just a hypothesis.
Both scientists are working towards FDA approval for clinical trials.
"It's exciting," Shepard says. "My [research] group tries to figure out ways that modern electronics technology can be put to work beyond applications like your cell phone and computer. Thinking how this will actually go into a human being, it's exciting."
CONTENT PROVIDED BY
BrainFacts/SfN
Discussion Questions
1) What is the “Dobelle Eye” and what advances are scientists hoping to gain in visual prosthesis?
2) How do people with vision impairments navigate using sound?
Also In Vision
Trending
Popular articles on BrainFacts.org