Sustaining the Sleep-Wake Cycle
- Reviewed15 Aug 2022
- Author Melissa Galinato
- Source BrainFacts/SfN
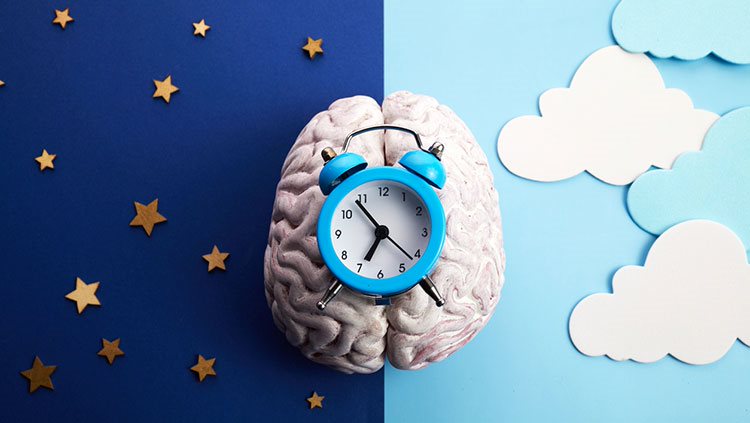
As the Earth journeys around the sun, it spins itself around its axis on a 24-hour schedule — dictating day and night on our planet. The 24-hour cycle defining a day on Earth also dictates our internal body clocks, oscillating us from sleep to wakefulness and back again.
Two main factors drive your body to crave sleep: the time of day or night (circadian system) and how long you have been awake (homeostatic system). The homeostatic and circadian systems are separate and act independently.
The circadian timing system is regulated by the suprachiasmatic nucleus, a small group of nerve cells in the hypothalamus functioning as a master clock. These cells express “clock proteins,” which go through a biochemical cycle of about 24 hours, setting the pace for daily cycles of activity, sleep, hormone release, and other bodily functions. The master clock neurons also receive input directly from the retina of the eye. As a result light can reset the master clock, adjusting it to the outside world’s day/night cycle — this explains how your sleep cycles can shift when you change time zones during travel. In addition, the suprachiasmatic nucleus sends signals through different brain regions, eventually contacting the VLPO and the orexin neurons in the lateral hypothalamus, which directly regulate arousal.
What happens in the brain when we don’t get enough sleep? The second system that regulates sleepiness is the homeostatic system, which makes you feel sleepy if you stay awake longer than usual. One important sleep factor is a chemical in the brain called adenosine. When you stay awake for a long time, adenosine levels in the brain increase. The increased adenosine binds to specific receptors on nerve cells in arousal centers slowing cellular activity and reducing arousal. Adenosine can increase the number of slow waves during SWS. As you get more sleep, adenosine levels fall and slow waves decrease in number. Caffeine acts as a stimulant by binding to adenosine receptors throughout the brain and preventing their interaction with adenosine. As a result, in the presence of caffeine, fewer receptors are available for the slowing influence of adenosine.
People often say they need to “catch up on sleep.” But can you really make up for lost sleep? Normally, the homeostatic and circadian systems act in a complementary fashion to produce a normal 24-hour cycle of sleep and wakefulness. Nonetheless, activating the brain’s arousal system can keep us awake even after a long period of wakefulness — for example, a late-night study session to prepare for an important exam. In normal circumstances, the homeostatic system will respond to the loss of sleep by increasing the duration of ensuing sleep and increasing the number of slow waves during the SWS episodes. Correlating with the time spent away, the rebound in slow wave activity is mediated by adenosine.
Adapted from the 8th edition of Brain Facts by Melissa Galinato.
CONTENT PROVIDED BY
BrainFacts/SfN
References
Allan, H. J., & Robert, M. (1997). The brain as a dream state generator: an activation-synthesis hypothesis of the dream process. Am J Psychiatr, 134, 1335-1348. https://pdfs.semanticscholar.org/f1af/886bfac2ee058ddaf1a6fb61dabe08e19b08.pdf
Becchetti, A., & Amadeo, A. (2016). Why we forget our dreams: Acetylcholine and norepinephrine in wakefulness and REM sleep. Behavioral and Brain Sciences, 39. https://www.cambridge.org/core/journals/behavioral-and-brain-sciences/article/div-classtitlewhy-we-forget-our-dreams-acetylcholine-and-norepinephrine-in-wakefulness-and-rem-sleepdiv/9C71B973B2BE9F117C17042BC0B43E7E
Carskadon, M.A., & Dement, W.C. (2011). Monitoring and staging human sleep. In M.H. Kryger, T. Roth, & W.C. Dement (Eds.), Principles and practice of sleep medicine, 5th edition, (pp 16-26). St. Louis: Elsevier Saunders. https://www.ninds.nih.gov/Disorders/Patient-Caregiver-Education/Fact-Sheets/Narcolepsy-Fact-Sheet
Chemelli, R. M., Willie, J. T., Sinton, C. M., Elmquist, J. K., Scammell, T., Lee, C. ... & Fitch, T. E. (1999). Narcolepsy in orexin knockout mice: molecular genetics of sleep regulation. Cell, 98(4), 437-451. http://www.sciencedirect.com/science/article/pii/S009286740081973X
Konadhode, R. R., Pelluru, D., & Shiromani, P. J. (2014). Neurons containing orexin or melanin concentrating hormone reciprocally regulate wake and sleep. Frontiers in systems neuroscience, 8. https://www.ncbi.nlm.nih.gov/pmc/articles/PMC4287014/
McEwen, B. S., & Karatsoreos, I. N. (2015). Sleep deprivation and circadian disruption: stress, allostasis, and allostatic load. Sleep medicine clinics, 10(1), 1-10. http://www.sciencedirect.com/science/article/pii/S1556407X14001246
Pelluru, D., Konadhode, R., & Shiromani, P. J. (2013). MCH neurons are the primary sleep-promoting group. Sleep, 36(12), 1779-1781. https://academic.oup.com/sleep/article/36/12/1779/2709396/MCH-Neurons-Are-the-Primary-Sleep-Promoting-Group
Schwartz, M. D., & Kilduff, T. S. (2015). The neurobiology of sleep and wakefulness. Psychiatric Clinics of North America, 38(4), 615-644. http://europepmc.org/articles/pmc4660253
Shiromani, P., Konadhod, R., Pelluru, D., Blanco-Centurion, C., Liu, M., & Mulholland, P. (2013). Optogenetic activation of specific neurons to ameliorate symptoms of narcolepsy in mice. Sleep Medicine, 14, e46-e47. http://www.sciencedirect.com/science/article/pii/S1389945713012896
Tsunematsu, T., Tabuchi, S., Tanaka, K. F., Boyden, E. S., Tominaga, M., & Yamanaka, A. (2013). Long-lasting silencing of orexin/hypocretin neurons using archaerhodopsin induces slow-wave sleep in mice. Behavioural brain research, 255, 64-74. http://www.sciencedirect.com/science/article/pii/S0166432813002921
Verweij, I. M., Romeijn, N., Smit, D. J., Piantoni, G., Van Someren, E. J., & van der Werf, Y. D. (2014). Sleep deprivation leads to a loss of functional connectivity in frontal brain regions. BMC neuroscience, 15(1), 88. https://bmcneurosci.biomedcentral.com/articles/10.1186/1471-2202-15-88
What to Read Next
Also In Sleep
Trending
Popular articles on BrainFacts.org