Shifting Brain Activity During Shut-Eye
- Reviewed15 Aug 2022
- Author Melissa Galinato
- Source BrainFacts/SfN
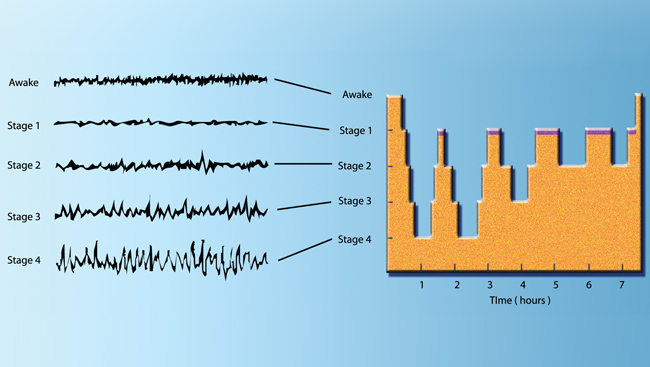
How many hours of sleep do you get every night? Most people spend one-third of their lives asleep. While that might appear to be a lot of time spent doing nothing, our brains are far from dormant as we rest each night. Brain activity during sleep is vital for brain health and for solidifying memories.
We know this because scientists can measure the brain’s electrical activity using electroencephalography (EEG). Electrodes attached to the scalp detect and record the net electrical activity of hundreds of thousands of cortical nerve cells. When a neuron is active, ions move in and out of the cell, altering the electrical charge across the cell membrane. An EEG detects the net electrical charge produced when neurons increase and decrease their activity as a group, in synchrony. The results are “brain waves” — the cyclic rising and falling of brain activity that can be important indicators of brain function. In sleep studies, scientists now recognize two main states: slow wave sleep (SWS) and rapid eye movement sleep (REM).
SWS gets its name from the high amplitude, low frequency, brain waves in EEG recordings. The high amplitude of slow waves indicates that many cortical neurons are switching their activity in a synchronized way from a depolarized (more excitable) state to a hyperpolarized (less excitable) state and back again. These slow waves appear to be important to sleep function — the longer a person stays awake, the slower waves they will experience during the SWS state. Slow waves become less frequent the longer the person is asleep. If awakened during SWS, most people recall only fragmented thoughts, not active dreams.
Have you ever seen a cat dreaming — twitching its whiskers or paws while it sleeps? Dreaming happens mainly during REM sleep, which takes its name from the periodic rapid eye movements people make in this state. Brain activity recorded during REM looks very similar to EEGs recorded while awake. EEG waves during REM sleep have much lower amplitudes than the SWS slow waves because neuron activity is less synchronized — some nerve cells depolarize while others hyperpolarize, and the “sum” of their electrical states is less positive (or negative) than if they acted in synchrony. Paradoxically, the fast, waking-like EEG activity during REM sleep is accompanied by atonia, a loss of muscle tone causing the body to become temporarily paralyzed. The only muscles remaining active are those that enable breathing and control eye movements. Oddly enough, the neurons of our motor cortex fire as rapidly during REM sleep as they do during waking movement — a fact that explains why movements like a kitten’s twitching paws can coincide with dreams.
During the night, periods of SWS and REM sleep alternate in 90-minute cycles with 75—80 minutes of SWS followed by 10–15 minutes of REM sleep. This cycle repeats, typically with deeper and longer periods of REM sleep towards morning. To study sleep disorders, researchers often use mice that have sleep structures qualitatively very similar to humans; however, rodents have shorter and more frequent sleep episodes lasting 3–30 minutes (sometimes longer). Rodents also sleep more during the day and are more active at night. Compare that to human adults, who are typically more active during the day and have one sleep episode at night lasting about 8 hours.
Adapted from the 8th edition of Brain Facts by Melissa Galinato.
CONTENT PROVIDED BY
BrainFacts/SfN
References
Allan, H. J., & Robert, M. (1997). The brain as a dream state generator: an activation-synthesis hypothesis of the dream process. Am J Psychiatr, 134, 1335-1348. https://pdfs.semanticscholar.org/f1af/886bfac2ee058ddaf1a6fb61dabe08e19b08.pdf
Becchetti, A., & Amadeo, A. (2016). Why we forget our dreams: Acetylcholine and norepinephrine in wakefulness and REM sleep. Behavioral and Brain Sciences, 39. https://www.cambridge.org/core/journals/behavioral-and-brain-sciences/article/div-classtitlewhy-we-forget-our-dreams-acetylcholine-and-norepinephrine-in-wakefulness-and-rem-sleepdiv/9C71B973B2BE9F117C17042BC0B43E7E
Carskadon, M.A., & Dement, W.C. (2011). Monitoring and staging human sleep. In M.H. Kryger, T. Roth, & W.C. Dement (Eds.), Principles and practice of sleep medicine, 5th edition, (pp 16-26). St. Louis: Elsevier Saunders. https://www.ninds.nih.gov/Disorders/Patient-Caregiver-Education/Fact-Sheets/Narcolepsy-Fact-Sheet
Chemelli, R. M., Willie, J. T., Sinton, C. M., Elmquist, J. K., Scammell, T., Lee, C. ... & Fitch, T. E. (1999). Narcolepsy in orexin knockout mice: molecular genetics of sleep regulation. Cell, 98(4), 437-451. http://www.sciencedirect.com/science/article/pii/S009286740081973X
Konadhode, R. R., Pelluru, D., & Shiromani, P. J. (2014). Neurons containing orexin or melanin concentrating hormone reciprocally regulate wake and sleep. Frontiers in systems neuroscience, 8. https://www.ncbi.nlm.nih.gov/pmc/articles/PMC4287014/
McEwen, B. S., & Karatsoreos, I. N. (2015). Sleep deprivation and circadian disruption: stress, allostasis, and allostatic load. Sleep medicine clinics, 10(1), 1-10. http://www.sciencedirect.com/science/article/pii/S1556407X14001246
Pelluru, D., Konadhode, R., & Shiromani, P. J. (2013). MCH neurons are the primary sleep-promoting group. Sleep, 36(12), 1779-1781. https://academic.oup.com/sleep/article/36/12/1779/2709396/MCH-Neurons-Are-the-Primary-Sleep-Promoting-Group
Schwartz, M. D., & Kilduff, T. S. (2015). The neurobiology of sleep and wakefulness. Psychiatric Clinics of North America, 38(4), 615-644. http://europepmc.org/articles/pmc4660253
Shiromani, P., Konadhod, R., Pelluru, D., Blanco-Centurion, C., Liu, M., & Mulholland, P. (2013). Optogenetic activation of specific neurons to ameliorate symptoms of narcolepsy in mice. Sleep Medicine, 14, e46-e47. http://www.sciencedirect.com/science/article/pii/S1389945713012896
Tsunematsu, T., Tabuchi, S., Tanaka, K. F., Boyden, E. S., Tominaga, M., & Yamanaka, A. (2013). Long-lasting silencing of orexin/hypocretin neurons using archaerhodopsin induces slow-wave sleep in mice. Behavioural brain research, 255, 64-74. http://www.sciencedirect.com/science/article/pii/S0166432813002921
Verweij, I. M., Romeijn, N., Smit, D. J., Piantoni, G., Van Someren, E. J., & van der Werf, Y. D. (2014). Sleep deprivation leads to a loss of functional connectivity in frontal brain regions. BMC neuroscience, 15(1), 88. https://bmcneurosci.biomedcentral.com/articles/10.1186/1471-2202-15-88