The Search for the Engram: Where Memory Lives in the Brain
- Published22 Oct 2020
- Author Mirjam Guesgen
- Source BrainFacts/SfN
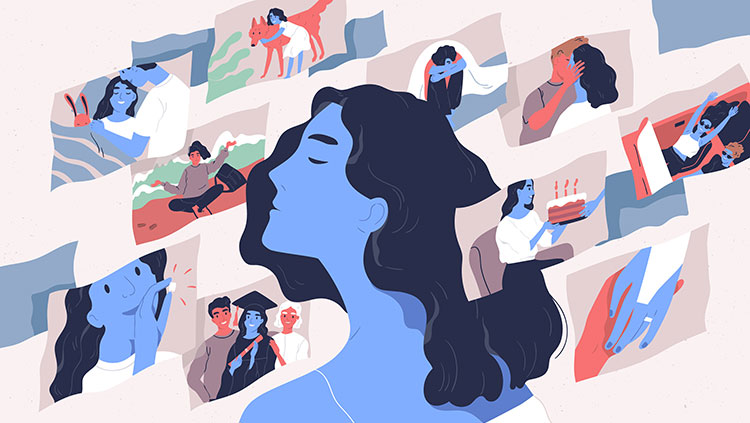
A mottled-grey sea slug floats in a small, clear container. Trunk-like tubes and antennae jut out of the gelatinous creature’s front end. A soft, flexible probe reaches down and gently prods the animal, which responds by tucking in one of those little tubes. Forty seconds later, the sea slug is still motionless, tube tucked in close, in a learned defense reaction. And yet, the sea slug had no reason to know the probe was dangerous. That knowledge — the memories of lived experience — came from a neighbor in the tank next door.
The memories were transferred the day before when a member of David Glanzman’s lab injected tiny snippets of genetic material from another sea slug that had been trained with mild shocks to take a defensive posture.
Glanzman, a neuroscientist at the University of California, Los Angeles, is trying to figure out how and where the brain stores memories. He hopes to show that memories are not just an abstract concept but are in fact a physical entity called an engram. It exists, his team believes, in the molecular code found deep within brain cells.
“I realized that our data indicated that an old hypothesis of memory, long discarded by neuroscientists, was probably correct,” he says.
Glanzman is not alone in his quest for the engram. Neuroscientists have been hunting for it for more than 100 years. Finding it would answer philosophical and scientific questions about our minds and bodies stretching all the way back to the first century. In many ways, our memories are us. They are our experiences, our history, our feelings. Isolating them is akin to isolating the essence of a person. Identifying the engram could also make strides toward restoring memory in people with memory disorders like Alzheimer’s.
Storing Memories in Synapses
Finding the engram, or even knowing where in the brain to look, is understandably difficult. The search begins in animals with simpler nervous systems, like the sea slug Aplysia californica. The idea is: Look at an animal’s brain, teach them something, and look for changes in the brain’s structure.
Aplysia reflexively withdraws its siphon when it’s poked. If you give it a mild electric shock at the same time, it learns to hold this defensive posture even longer. An innocuous poke later will trigger the same, sensitized response. Getting this memory to stick — a process called consolidation — requires repeated poking and shocking sessions, much like building muscles takes several trips to the gym.
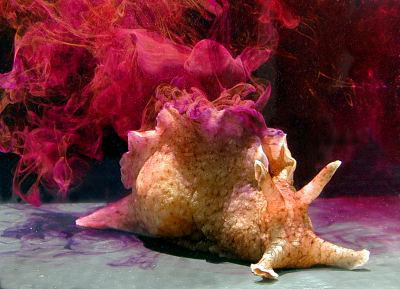
By mimicking these experiments using neurons in a dish, neuroscientists learned memory consolidation strengthens the connections between neurons: the tiny branches at the ends of neurons reach out and link up with neighboring neurons. “You can actually see the growth right there in the petri dish,” Glanzman says. This led to the popular theory that memories reside in the connections between neurons, or synapses.
Recalling a memory triggers a new round of consolidation, called reconsolidation. Both processes depend on neurons making new proteins to support and sustain the growth. If, for instance, researchers block protein synthesis by injecting a protein inhibitor into animals’ brains shortly after training, memories fail to take hold.
But Glanzman wanted to know what happens if reconsolidation is blocked. So, he popped a simple network of Aplysia neurons in a dish. Instead of poking, the neurons got several doses of serotonin, the neurotransmitter released when a sea slug gets a tail shock.
He could tell the neurons had “learned” because they formed new synapses. When Glanzman swirled in a “reminder” dose of serotonin plus a protein inhibitor, the new synapses retreated again.
But it wasn’t just the new synapses that retreated — synapses that sprouted after the training and others that were already there were both pared back. “I really had trouble wrapping my head around this,” Glanzman says. This suggested to him that the engram might not be in synapses after all.
The Nuclear Theory
Glanzman took a cue from researchers who were studying the epigenetic changes behind memory, or changes in how the genetic code is expressed. He repeated the Aplysia experiments, except this time he blocked DNA methylation, a process where carbon and hydrogen atoms attach to DNA and change the way genes are expressed. Just like before, blocking methylation during or shortly after behavioral training prevented memory formation. But strangely, it also erased memories that had already been consolidated. When they injected the methylation blockers weeks after the sea slugs had learned to withdraw their siphons, they suddenly forgot.
The experiment led Glanzman and his colleagues to their latest theory: memory is found in the neuron’s nucleus, in bits of genetic material called non-coding RNA helping to switch genes on and off. “The way I look at it is that the synapses are like the hands of a concert pianist,” Glanzman explains. “Without the hands, the concert pianist can’t play Mozart. But it’s not because they don’t have the knowledge; they don’t have the ability to express their knowledge.” Blocking protein synthesis immediately after training erases the animal’s way of expressing a memory, Glanzman says, but blocking DNA methylation erases the memory itself.
And it seems the theory has some weight, as his team demonstrated they could transfer memories between sea slugs by transferring non-coding RNA. “If memories were stored in the synapses, there is no way our experiment would have worked,” Glanzman says.
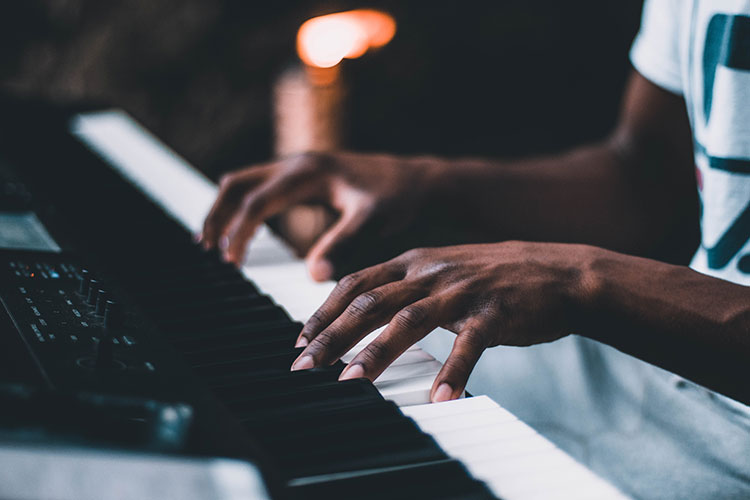
If the nuclear theory is correct, it could hold exciting possibilities for reversing memory disorders, like Alzheimer’s, where synapses are destroyed. “If our idea is right, maybe the memories aren’t really gone. The memories could be there but locked in the nucleus and unable to be expressed. If we could figure out a way to restore the synapses then we could potentially restore the memory,” Glanzman says.
Of course, a sea slug’s defensive reflex bears little resemblance to the sensory-rich narratives of human memory. “Memories in people are likely much more complex,” says Sheena Josselyn, a neuroscientist at the Hospital for Sick Children in Toronto. Some of the basic building blocks might be the same, “but the human brain contains many more neurons and a great deal of complexity in its wiring,” she says.
It’s important to replicate the intriguing findings in other species and see if the same holds true for other kinds of memories, she adds. “But the results are incredibly exciting and certainly should be followed up.”
Glanzman admits the team didn’t transfer a complex memory, like remembering when you got a bike for your fifth birthday, but it’s a start. They’re now attempting to replicate the Aplysia experiment in rats and eventually hope to discover exactly which bits of non-coding RNA are the memory. “If we can find those, we can get an idea of the kind of nuclear change they’re producing,” Glanzman says.
Connecting the Dots
Not everyone is convinced that epigenetic change is the final answer to the engram puzzle.
“I find [the nuclear model] to be completely implausible,” says Tomás Ryan, an associate neuroscience professor at Trinity College Dublin. DNA methylation is necessary for normal gene expression, not just forming memories. “It’s not surprising that if you screw up these things, you’re going to get an effect on memory [too],” he says.
Further, changing what’s going on in the nucleus doesn’t match the timescale of memory formation or recall, he adds. Animals can recall a short-term memory seconds after learning it, meaning it must be stored (at least temporarily) somewhere in the brain. But sending information to the neuron’s nucleus and changing how genes are expressed takes much longer, Ryan says. “In the quickest instance you’re talking ten to 15 minutes or hours.”
Ryan and his colleagues believe memory is stored as a network or pathway of connections between neurons. It’s a subtle distinction from the idea that memory resides in synapses. “Yes, a circuit requires specific synaptic connections, but I don’t think the synaptic connections on their own are carrying the information. The information is in the shape of the circuit.” It’s like a connect-the-dots picture — you see the image by looking at it in its entirety, rather than focusing on the individual dots and the lines connecting them.
Ryan and his team have found some evidence for this theory. They trained mice to freeze at the sound of a tone by first pairing it with an electric shock. Injecting the mice with protein inhibitors blocked memory retrieval — the mice no longer froze when they heard the tone. But, the researchers found they could reactivate the memory by stimulating particular neurons using light, a technique called optogenetics. The memory wasn’t lost when the individual synapses were lost; it somehow persisted in the pattern of connections between neurons.
Two Sides of the Same Coin
From epigenetics to synapses to circuits, there are lots of competing theories about the engram. Which is correct? The answer might be all of them.
The theories “are not as much in conflict as they’re portrayed to be,” says computational neuroscientist Kanaka Rajan. For instance, creating and maintaining new synapses requires production of new proteins, which are coded for by genes. “An engram encoded by synapses may to some extent also be encoded in genetics,” Rajan says. The same goes for the circuit and epigenetic theories. “I think they may be along the same continuum or two sides of the same coin,” she says.
Her lab at the Icahn School of Medicine at Mount Sinai studies how computational and mathematical models can help us understand how the brain works. The approach “gives you the ability to see the greater patterns, beyond the details of one experiment,” she says.
Such an approach might be particularly fitting for the engram. “I think we need to step back from our pet hypothesis and acknowledge that maybe many things are happening when a memory is stored and retrieved,” Josselyn says. It’s probably not as simple as one molecule or one pathway, she says.
Glanzman knows the field has a long way to go. “Until we can identify the precise changes that underlie a specific long-term memory, any theory is going to be a hard sell,” Glanzman says.
CONTENT PROVIDED BY
BrainFacts/SfN
References
Bédécarrats, A., Chen, S., Pearce, K., Cai, D., & Glanzman, D. L. (2018). RNA from Trained Aplysia Can Induce an Epigenetic Engram for Long-Term Sensitization in Untrained Aplysia. ENeuro, 5(3). doi: 10.1523/ENEURO.0038-18.2018
Bi, G., & Poo, M. (1999). Distributed synaptic modification in neural networks induced by patterned stimulation. Nature, 401(6755), 792–796. doi: 10.1038/44573
Chen, S., Cai, D., Pearce, K., Sun, P. Y.-W., Roberts, A. C., & Glanzman, D. L. (2014). Reinstatement of long-term memory following erasure of its behavioral and synaptic expression in Aplysia. ELife, 3, e03896. doi: 10.7554/eLife.03896
Ezzeddine, Y., & Glanzman, D. L. (2003). Prolonged Habituation of the Gill-Withdrawal Reflex in Aplysia Depends on Protein Synthesis, Protein Phosphatase Activity, and Postsynaptic Glutamate Receptors. Journal of Neuroscience, 23(29), 9585–9594. doi: 10.1523/JNEUROSCI.23-29-09585.2003
Liu, X., Ramirez, S., Pang, P. T., Puryear, C. B., Govindarajan, A., Deisseroth, K., & Tonegawa, S. (2012). Optogenetic stimulation of a hippocampal engram activates fear memory recall. Nature, 484(7394), 381–385. doi: 10.1038/nature11028
Pearce, K., Cai, D., Roberts, A. C., & Glanzman, D. L. (2017). Role of protein synthesis and DNA methylation in the consolidation and maintenance of long-term memory in Aplysia. ELife, 6, e18299. doi: 10.7554/eLife.18299
Poo, M.-M., Pignatelli, M., Ryan, T. J., Tonegawa, S., Bonhoeffer, T., Martin, K. C., … Stevens, C. (2016). What is memory? The present state of the engram. BMC Biology, 14, 40. doi: 10.1186/s12915-016-0261-6
Queenan, B. N., Ryan, T. J., Gazzaniga, M. S., & Gallistel, C. R. (2017). On the research of time past: The hunt for the substrate of memory. Annals of the New York Academy of Sciences, 1396(1), 108–125. doi: 10.1111/nyas.13348
Ryan, T. J., Roy, D. S., Pignatelli, M., Arons, A., & Tonegawa, S. (2015). Engram cells retain memory under retrograde amnesia. Science, 348(6238), 1007–1013. doi: 10.1126/science.aaa5542
Scoville, W. B., & Milner, B. (1957). Loss of Revent Memory After Bilateral Hippocampal Lesions. Journal of Neurology, Neurosurgery, and Psychiatry, 20(1), 11–21. Retrieved from: https://www.ncbi.nlm.nih.gov/pmc/articles/PMC497229/
Squire, L. R. (2004). Memory systems of the brain: A brief history and current perspective. Neurobiology of Learning and Memory, 82(3), 171–177. doi: 10.1016/j.nlm.2004.06.005
Thompson, R. F. (1986). The neurobiology of learning and memory. Science, 233(4767), 941–947. doi: 10.1126/science.3738519
Yokose, J., Okubo-Suzuki, R., Nomoto, M., Ohkawa, N., Nishizono, H., Suzuki, A., … Inokuchi, K. (2017). Overlapping memory trace indispensable for linking, but not recalling, individual memories. Science, 355(6323), 398–403. doi: 10.1126/science.aal2690
Zhang, F., Wang, L.-P., Brauner, M., Liewald, J. F., Kay, K., Watzke, N., … Deisseroth, K. (2007). Multimodal fast optical interrogation of neural circuitry. Nature, 446(7136), 633–639. doi: 10.1038/nature05744