Measuring Neural Physiology
- Reviewed27 Feb 2023
- Author Susan Rojahn
- Source BrainFacts/SfN
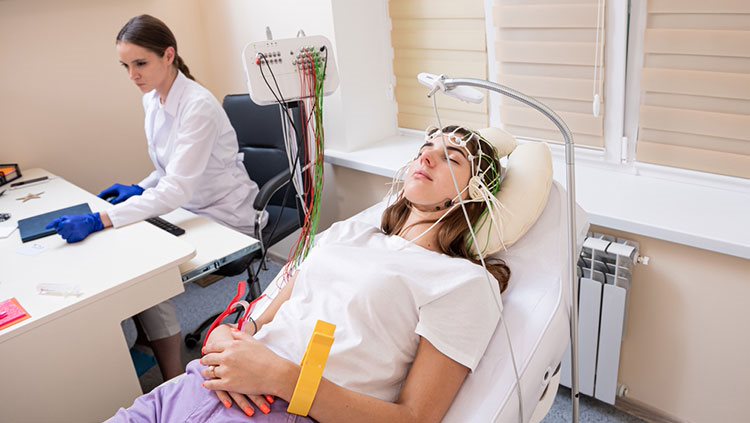
Brains act as centralization hubs that process and govern everything we experience and do. To do this is no easy feat, and we are steadily coming to better understand how we carry out this major undertaking.
Information is conveyed along the neuronal pathways that crisscross through our brains as electrical activity traveling down axons. To study this activity, researchers measure changes in the electrical charge of individual neurons using techniques of electrophysiology. A thin glass electrode is placed inside a neuron to measure the voltage across its cell membrane, which changes when the neuron is activated. This technique can measure neuronal activity inside the brains of living lab animals such as rats or mice, enabling scientists to study how neurons transmit electrical information in their normal physical context. Alternatively, a slice of brain can be kept “alive” for a short time in a Petri dish, if the right environment (temperature, pH, ion concentrations, etc.) is provided. In an isolated brain slice, researchers can better identify the exact cell they are recording from and can infuse drugs into the Petri dish to determine their effects on the brain.
Using these methods, scientists have made critical discoveries about synaptic plasticity — the capacity of a synapse to become stronger or weaker in response to sensory inputs or other activity. For example, repeatedly stimulating a neuron by training an animal in a particular task, or by direct electrical stimulation, increases the synaptic strength and the chance that the downstream neuron will react to the incoming signal.
A disadvantage of electrophysiology, as described above, is that the techniques are highly invasive. However, another method, called electroencephalography or EEG, is able to record human brain activity without invasive or harmful procedures. In EEG, about 20 thin metal discs are placed on the scalp. Each disk is connected by thin wires to a machine that records the activity of neurons near the brain surface. This approach has been especially useful for understanding epilepsy and the stages of sleep. However, it does not provide information at the level of individual neurons.
Researchers who need to look at individual neurons in a living brain can use a technique called two-photon microscopy. A lab animal such as a fly or mouse must be genetically modified so that some of its neurons produce a protein that glows when a laser beam shines on them. Two-photon microscopy has enabled scientists to understand changes in the brain during normal processes like learning, as well as changes that occur over the course of a disease — for example, watching how the branches on neurons near Alzheimer’s-like plaques break down over time.
Adapted from the 8th edition of Brain Facts by Susan Rojahn.
CONTENT PROVIDED BY
BrainFacts/SfN
References
Berman, M. G., Jonides, J., & Nee, D. E. (2006). Studying Mind and Brain with fMRI. Social cognitive and affective neuroscience, 1(2), 158–161. https://doi.org/10.1093/scan/nsl019
Bögershausen, N., & Wollnik, B. (2013). Unmasking Kabuki syndrome. Clinical genetics, 83(3), 201–211. https://doi.org/10.1111/cge.12051
Boyden E. S. (2015). Optogenetics and the Future of Neuroscience. Nature neuroscience, 18(9), 1200–1201. https://doi.org/10.1038/nn.4094
Caraci, F., Leggio, G. M., Salomone, S., & Drago, F. (2017). New Drugs in Psychiatry: Focus on New Pharmacological Targets. F1000Research, 6, 397. https://doi.org/10.12688/f1000research.10233.1
Carter M. and Shieh J. C. (2015). Guide to Research Techniques in Neuroscience. Academic Press. p 164.
Carter N. P. (2007). Methods and Strategies for Analyzing Copy Number Variation Using DNA Microarrays. Nature genetics, 39(7 Suppl), S16–S21. https://doi.org/10.1038/ng2028
Chefer, V. I., Thompson, A. C., Zapata, A., & Shippenberg, T. S. (2009). Overview of Brain Microdialysis. Current protocols in neuroscience, Chapter 7, Unit 7.1. https://doi.org/10.1002/0471142301.ns0701s47
Clancy, S. (2008). Copy Number Variation. Nature Education, 1(1):95. https://www.nature.com/scitable/topicpage/copy-number-variation-445/
Cohen M. X. (2017). Where Does EEG Come From and What Does It Mean?. Trends in neurosciences, 40(4), 208–218. https://doi.org/10.1016/j.tins.2017.02.004
Courtney, K. E., & Ray, L. A. (2014). Methamphetamine: An Update on Epidemiology, Pharmacology, Clinical Phenomenology, and Treatment Literature. Drug and alcohol dependence, 143, 11–21. https://doi.org/10.1016/j.drugalcdep.2014.08.003
Cui, X., Bray, S., Bryant, D. M., Glover, G. H., & Reiss, A. L. (2011). A Quantitative Comparison of NIRS and fMRI Across Multiple Cognitive Tasks. NeuroImage, 54(4), 2808–2821. https://doi.org/10.1016/j.neuroimage.2010.10.069
Flagel, S. B., Chaudhury, S., Waselus, M., Kelly, R., Sewani, S., Clinton, S. M., Thompson, R. C., Watson, S. J., Jr, & Akil, H. (2016). Genetic Background and Epigenetic Modifications in the Core of the Nucleus Accumbens Predict Addiction-like Behavior in a Rat Model. Proceedings of the National Academy of Sciences of the United States of America, 113(20), E2861–E2870. https://doi.org/10.1073/pnas.1520491113
Gratten, J., Wray, N. R., Keller, M. C., & Visscher, P. M. (2014). Large-scale genomics unveils the genetic architecture of psychiatric disorders. Nature neuroscience, 17(6), 782–790. https://doi.org/10.1038/nn.3708
Hämäläinen, M., Hari, R., Ilmoniemi, R. J., Knuutila, J., & Lounasmaa, O. V. (1993). Magnetoencephalography—Theory, Instrumentation, and Applications to Noninvasive Studies of the Working Human Brain. Reviews of modern Physics, 65(2), 413. https://journals.aps.org/rmp/abstract/10.1103/RevModPhys.65.413
Hanrieder, J., Phan, N. T., Kurczy, M. E., & Ewing, A. G. (2013). Imaging Mass Spectrometry in Neuroscience. ACS chemical neuroscience, 4(5), 666–679. https://doi.org/10.1021/cn400053c
Heather, J. M., & Chain, B. (2016). The Sequence of Sequencers: The History of Sequencing DNA. Genomics, 107(1), 1–8. https://doi.org/10.1016/j.ygeno.2015.11.003
Heidenreich, M., & Zhang, F. (2016). Applications of CRISPR-Cas Systems in Neuroscience. Nature reviews. Neuroscience, 17(1), 36–44. https://doi.org/10.1038/nrn.2015.2
Herbst, S. M., Proepper, C. R., Geis, T., Borggraefe, I., Hahn, A., Debus, O., Haeussler, M., von Gersdorff, G., Kurlemann, G., Ensslen, M., Beaud, N., Budde, J., Gilbert, M., Heiming, R., Morgner, R., Philippi, H., Ross, S., Strobl-Wildemann, G., Muelleder, K., Vosschulte, P., … Hehr, U. (2016). LIS1-associated Classic Lissencephaly: A Retrospective, Multicenter Survey of the Epileptogenic Phenotype and Response to Antiepileptic Drugs. Brain & development, 38(4), 399–406. https://doi.org/10.1016/j.braindev.2015.10.001
Hopf, F. W., & Lesscher, H. M. (2014). Rodent Models for Compulsive Alcohol Intake. Alcohol (Fayetteville, N.Y.), 48(3), 253–264. https://doi.org/10.1016/j.alcohol.2014.03.001
Johnson, A. C., & Greenwood-Van Meerveld, B. (2016). The Pharmacology of Visceral Pain. Advances in pharmacology (San Diego, Calif.), 75, 273–301. https://doi.org/10.1016/bs.apha.2015.11.002
Kandel, E. R., Dudai, Y., & Mayford, M. R. (2014). The Molecular and Systems Biology of Memory. Cell, 157(1), 163–186. https://doi.org/10.1016/j.cell.2014.03.001
Lee, G. J., Park, J. H., & Park, H. K. (2008). Microdialysis Applications in Neuroscience. Neurological research, 30(7), 661–668. https://doi.org/10.1179/174313208X289570
Leroy, A., Foucher, J. R., Pins, D., Delmaire, C., Thomas, P., Roser, M. M., Lefebvre, S., Amad, A., Fovet, T., Jaafari, N., & Jardri, R. (2017). fMRI Capture of Auditory Hallucinations: Validation of the Two-Steps Method. Human brain mapping, 38(10), 4966–4979. https://doi.org/10.1002/hbm.23707
Liu, Z., Ding, L., & He, B. (2006). Integration of EEG/MEG with MRI and fMRI. IEEE engineering in medicine and biology magazine : the quarterly magazine of the Engineering in Medicine & Biology Society, 25(4), 46–53. https://doi.org/10.1109/memb.2006.1657787
Lodish H., Berk A., Zipurksy S. L. et. al., editors. (2000). Molecular Cell Biology, 4th edition. Freeman, p 94, 140, 147-148, 268-269.
Malik, A. N., Vierbuchen, T., Hemberg, M., Rubin, A. A., Ling, E., Couch, C. H., Stroud, H., Spiegel, I., Farh, K. K., Harmin, D. A., & Greenberg, M. E. (2014). Genome-wide Identification and Characterization of Functional Neuronal Activity-Dependent Enhancers. Nature neuroscience, 17(10), 1330–1339. https://doi.org/10.1038/nn.3808
Mayford, M., Siegelbaum, S. A., & Kandel, E. R. (2012). Synapses and Memory Storage. Cold Spring Harbor perspectives in biology, 4(6), a005751. https://doi.org/10.1101/cshperspect.a005751
Maze, I., Shen, L., Zhang, B., Garcia, B. A., Shao, N., Mitchell, A., Sun, H., Akbarian, S., Allis, C. D., & Nestler, E. J. (2014). Analytical Tools and Current Challenges in the Modern Era of Neuroepigenomics. Nature neuroscience, 17(11), 1476–1490. https://doi.org/10.1038/nn.3816
National Human Genome Research Institute. (July 2017). An Overview of the Human Genome Project. Accessed July 17, 2017 at https://www.genome.gov/12011238/an-overview-of-the-human-genome-project/
National Institute of Mental Health. (2017). Brain Stimulation Therapies. Accessed July 17, 2017 at https://www.nimh.nih.gov/health/topics/brain-stimulation-therapies/brain-stimulation-therapies.shtml
Olgiati, S., Quadri, M., & Bonifati, V. (2016). Genetics of Movement Disorders in the Next-Generation Sequencing Era. Movement disorders, 31(4), 458–470. https://doi.org/10.1002/mds.26521
Perry, R. H., Blessed, G., Perry, E. K., & Tomlinson, B. E. (1980). Histochemical Observations on Cholinesterase Activities in the Brains of Elderly Normal and Demented (Alzheimer-type) Patients. Age and ageing, 9(1), 9–16. https://doi.org/10.1093/ageing/9.1.9
Purves D, Augustine GJ, Fitzpatrick D, et al., editors. (2008). Neuroscience. 4th edition. Sinauer Associates, Inc. p 3-5, 16-17, 19-21, 25-27, 181-187, 465, 559, 673-674, 715-717.
Sejnowski, T. J., Koch, C., & Churchland, P. S. (1988). Computational Neuroscience. Science (New York, N.Y.), 241(4871), 1299–1306. https://doi.org/10.1126/science.3045969
Sokolowski M. B. (2001). Drosophila: Genetics Meets Behaviour. Nature reviews. Genetics, 2(11), 879–890. https://doi.org/10.1038/35098592
Svoboda, K., & Yasuda, R. (2006). Principles of Two-Photon Excitation Microscopy and its Applications to Neuroscience. Neuron, 50(6), 823–839. https://doi.org/10.1016/j.neuron.2006.05.019
Turek, F. W., Pinto, L. H., Vitaterna, M. H., Penev, P. D., Zee, P. C., & Takahashi, J. S. (1995). Pharmacological and Genetic Approaches for the Study of Circadian Rhythms in Mammals. Frontiers in neuroendocrinology, 16(3), 191–223. https://doi.org/10.1006/frne.1995.1007
US National Library of Medicine, National Institutes of Health. (2017). Genetics Home Reference – Huntington Disease. Accessed July 17, 2017 at https://ghr.nlm.nih.gov/condition/huntington-disease#genes
Usdin, K., & Kumari, D. (2015). Repeat-mediated Epigenetic Dysregulation of the FMR1 Gene in the Fragile X-related Disorders. Frontiers in genetics, 6, 192. https://doi.org/10.3389/fgene.2015.00192
Yoshino, K., Oka, N., Yamamoto, K., Takahashi, H., & Kato, T. (2013). Functional Brain Imaging Using Near-infrared Spectroscopy During Actual Driving on an Expressway. Frontiers in human neuroscience, 7, 882. https://doi.org/10.3389/fnhum.2013.00882