Analyzing Our Genetic Blueprints
- Reviewed6 Mar 2023
- Author Susan Rojahn
- Source BrainFacts/SfN
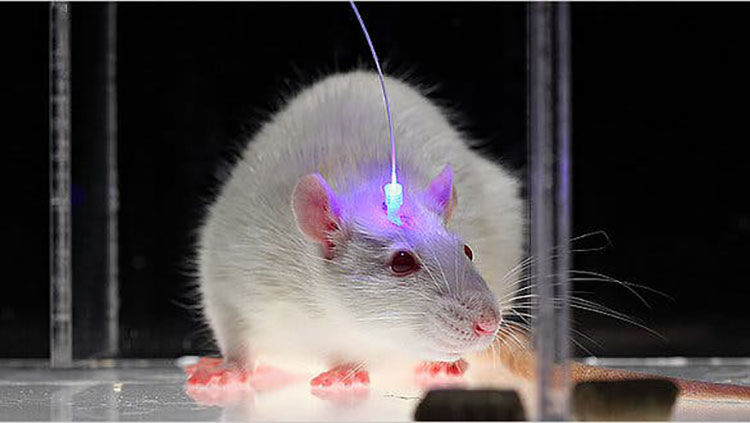
Found in nearly all our cells, our deoxyribonucleic acid (DNA) contains our genetic blueprints — the foundation which helps guide the creation and maintenance of everything we’re biologically made of.
The human genome is made up of 3 billion pairs of DNA letters or “bases.” This multitude of adenine (A), cytosine (C), guanine (G), and thymine (T) bases comprises an estimated 20,000 genes that spell out instructions for making proteins, along with regulatory and other non-coding DNA regions whose functions are not fully known. Scientists study genetics in many ways, such as following diseases or other traits through family pedigrees or identifying the exact order of DNA bases (the DNA “sequence”) that code for a given trait. More recent genetic tools enable scientists to manipulate genes and other genetic features to better understand how the brain works and how to treat it in cases of dysfunction or disease.
Scientists often don’t know which gene or other DNA feature controls a trait. At the outset, a particular trait could be encoded on any of the 23 pairs of chromosomes in a typical human cell. But with genetic linkage studies, researchers have begun to map gene locations. First, researchers must identify another trait with a known chromosomal location that tends to be inherited with or “linked” to the trait of interest. This technique, which narrows down the likely location of the gene of interest, is the first step toward identifying the genetic basis of many neurological disorders.
When you think about mutations, you probably think of harmful changes in one or several DNA bases within a gene. But some disorders result from an overabundance of copies or repeats of a stretch of DNA. This is the case with Huntington’s disease. The normal HTT gene has about a dozen repeats of a small stretch of DNA within the gene, but Huntington’s patients can have more than 100 of these repeats. Researchers now use DNA chips or microarrays to identify such variations in copy number. The “array” of a microarray refers to the thousands of spots arrayed in rows and columns on the surface of the chip; each spot contains a known DNA sequence or gene, which can grab onto corresponding bits of the genome being analyzed. Using this tool, scientists are able to compare DNA samples of two people, perhaps one healthy and one with a disorder, to see if certain pieces of DNA are repeated more in one person than in the other. Another type of microarray helps researchers determine if a patient has a chromosomal translocation — a chunk of a chromosome that has been misplaced onto another chromosome.
Recent years have seen great advances in DNA sequencing methods, allowing researchers to more efficiently and affordably explore the exact DNA sequence that might underlie brain disorders. In the early 2000s, the Human Genome Project made public much of the human genome sequence; in the years that followed, the science of genomics has enhanced scientists’ understanding of brain function at the level of genes, cells, and circuits. Genomics can help identify genetic variations that cause conditions ranging from depression to schizophrenia to movement disorders.
Genetics research now goes far beyond reading the sequence of bases in the genome. In the last few years, scientists have harnessed a molecular tool that can edit the genome more precisely and efficiently than was previously possible. This tool, called CRISPR (which stands for Clustered Regularly Interspaced Short Palindromic Repeats), evolved as a bacterial immune system that targets viral invaders. Scientists have harnessed CRISPR’s components to home in on specific DNA sequences in lab animals and human cell cultures. By tethering DNA-cutting enzymes to this targeting system, scientists can recreate mutations found in patients with neurological disorders, or even insert new bits of DNA to test their effect. With CRISPR, scientists have been able to mimic Alzheimer’s in rodents in order to study the disease and its potential treatments. CRISPR is also used to study mutated human neurons in Petri dishes. Researchers can observe how mutations that cause autism, Parkinson’s disease, or other conditions affect neuronal growth and function.
Optogenetics is another intersection of genetic tools with brain science. This technique allows researchers to control brain activity with flashes of light. Scientists genetically modify a lab animal, like a mouse, so that its neurons produce a light-responsive protein. Then, optical fibers are inserted into the brain to allow light to shine on those neurons — either activating or silencing them. Optogenetics has helped scientists better understand how neurons work together in circuits. This technique has also been used to control animal behaviors ranging from sleep to drug addiction.
Oddly enough, genetics is not always about genes. As mentioned above, much of the human genome contains DNA sequences that are not genes, whose job is to regulate gene activity. These regulatory sequences, and the enzymes that make changes to them, help determine under what conditions (in what cells, at what age, etc.) a gene is expressed or repressed. These epigenetic changes occur in cells when chemical tags are placed on the regulatory regions of certain genes; the tags influence whether those genes will be turned on or off. In the past decade, epigenetics research has begun to clarify the role of gene regulation in brain development and learning. Epigenetics has also revealed how mutations in the regulatory regions of DNA can cause disease, just as mutations can in genes.
Genetics in Neurological Diseases
The impact of mutations varies from person to person, and from disease to disease. A particular mutation might explain some cases of a disorder but not others, or it could be only one of several genetic changes affecting a patient. Lissencephaly is a brain malformation in which the surface of the brain is smooth, unlike normal brains whose surfaces have ridges and grooves. It affects development. Babies with lissencephaly start having spasms in the first months of life and develop drug-resistant epilepsy and severe intellectual and motor disabilities. Although about 70% of these patients have mutations in the LIS1 gene, at least two other mutations have been associated with the condition. Another complex genetic condition, Kabuki syndrome, is marked by intellectual disabilities, a distinctive facial appearance, slow growth in infancy, and other physical problems. Kabuki syndrome is hard to diagnose because some symptoms, such as intellectual disability, range from mild to severe. DNA sequencing has found that most patients, but not all, have mutations in the KMT2D gene — and some patients carry the mutations in only some of their cells. In addition, people with Kabuki syndrome may have mutations in other genes that function like KMT2D.
It is also possible for a person to carry a mutation but exhibit no outward signs. Fragile X syndrome, the most common form of congenital intellectual disability in males, is caused by an excessive number of DNA sequence (CGG) repeats within the FMR1 gene. The protein product of the FMR1 gene, which is important for synapse function, is disrupted by these repeats. While some people with elevated numbers of the sequence repeats may not be affected, they are carriers with a risk of passing it on to their children.
Adapted from the 8th edition of Brain Facts by Susan Rojahn.
CONTENT PROVIDED BY
BrainFacts/SfN
References
Bao, W., Jia, H., Finnema, S., Cai, Z., Carson, R. E., & Huang, Y. H. (2017). PET Imaging for Early Detection of Alzheimer's Disease: From Pathologic to Physiologic Biomarkers. PET clinics, 12(3), 329–350. https://doi.org/10.1016/j.cpet.2017.03.001
Berman, M. G., Jonides, J., & Nee, D. E. (2006). Studying Mind and Brain with fMRI. Social cognitive and affective neuroscience, 1(2), 158–161. https://doi.org/10.1093/scan/nsl019
Bögershausen, N., & Wollnik, B. (2013). Unmasking Kabuki syndrome. Clinical genetics, 83(3), 201–211. https://doi.org/10.1111/cge.12051
Boyden E. S. (2015). Optogenetics and the Future of Neuroscience. Nature neuroscience, 18(9), 1200–1201. https://doi.org/10.1038/nn.4094
Caraci, F., Leggio, G. M., Salomone, S., & Drago, F. (2017). New Drugs in Psychiatry: Focus on New Pharmacological Targets. F1000Research, 6, 397. https://doi.org/10.12688/f1000research.10233.1
Carter M. and Shieh J. C. (2015). Guide to Research Techniques in Neuroscience. Academic Press. p 164.
Carter N. P. (2007). Methods and Strategies for Analyzing Copy Number Variation Using DNA Microarrays. Nature genetics, 39(7 Suppl), S16–S21. https://doi.org/10.1038/ng2028
Chefer, V. I., Thompson, A. C., Zapata, A., & Shippenberg, T. S. (2009). Overview of Brain Microdialysis. Current protocols in neuroscience, Chapter 7, Unit 7.1. https://doi.org/10.1002/0471142301.ns0701s47
Clancy, S. (2008). Copy Number Variation. Nature Education, 1(1):95. https://www.nature.com/scitable/topicpage/copy-number-variation-445/
Cohen M. X. (2017). Where Does EEG Come From and What Does It Mean?. Trends in neurosciences, 40(4), 208–218. https://doi.org/10.1016/j.tins.2017.02.004
Courtney, K. E., & Ray, L. A. (2014). Methamphetamine: An Update on Epidemiology, Pharmacology, Clinical Phenomenology, and Treatment Literature. Drug and alcohol dependence, 143, 11–21. https://doi.org/10.1016/j.drugalcdep.2014.08.003
Cui, X., Bray, S., Bryant, D. M., Glover, G. H., & Reiss, A. L. (2011). A Quantitative Comparison of NIRS and fMRI Across Multiple Cognitive Tasks. NeuroImage, 54(4), 2808–2821. https://doi.org/10.1016/j.neuroimage.2010.10.069
Flagel, S. B., Chaudhury, S., Waselus, M., Kelly, R., Sewani, S., Clinton, S. M., Thompson, R. C., Watson, S. J., Jr, & Akil, H. (2016). Genetic Background and Epigenetic Modifications in the Core of the Nucleus Accumbens Predict Addiction-like Behavior in a Rat Model. Proceedings of the National Academy of Sciences of the United States of America, 113(20), E2861–E2870. https://doi.org/10.1073/pnas.1520491113
Gratten, J., Wray, N. R., Keller, M. C., & Visscher, P. M. (2014). Large-scale genomics unveils the genetic architecture of psychiatric disorders. Nature neuroscience, 17(6), 782–790. https://doi.org/10.1038/nn.3708
Hämäläinen, M., Hari, R., Ilmoniemi, R. J., Knuutila, J., & Lounasmaa, O. V. (1993). Magnetoencephalography—Theory, Instrumentation, and Applications to Noninvasive Studies of the Working Human Brain. Reviews of modern Physics, 65(2), 413. https://journals.aps.org/rmp/abstract/10.1103/RevModPhys.65.413
Hanrieder, J., Phan, N. T., Kurczy, M. E., & Ewing, A. G. (2013). Imaging Mass Spectrometry in Neuroscience. ACS chemical neuroscience, 4(5), 666–679. https://doi.org/10.1021/cn400053c
Heather, J. M., & Chain, B. (2016). The Sequence of Sequencers: The History of Sequencing DNA. Genomics, 107(1), 1–8. https://doi.org/10.1016/j.ygeno.2015.11.003
Heidenreich, M., & Zhang, F. (2016). Applications of CRISPR-Cas Systems in Neuroscience. Nature reviews. Neuroscience, 17(1), 36–44. https://doi.org/10.1038/nrn.2015.2
Herbst, S. M., Proepper, C. R., Geis, T., Borggraefe, I., Hahn, A., Debus, O., Haeussler, M., von Gersdorff, G., Kurlemann, G., Ensslen, M., Beaud, N., Budde, J., Gilbert, M., Heiming, R., Morgner, R., Philippi, H., Ross, S., Strobl-Wildemann, G., Muelleder, K., Vosschulte, P., … Hehr, U. (2016). LIS1-associated Classic Lissencephaly: A Retrospective, Multicenter Survey of the Epileptogenic Phenotype and Response to Antiepileptic Drugs. Brain & development, 38(4), 399–406. https://doi.org/10.1016/j.braindev.2015.10.001
Hopf, F. W., & Lesscher, H. M. (2014). Rodent Models for Compulsive Alcohol Intake. Alcohol (Fayetteville, N.Y.), 48(3), 253–264. https://doi.org/10.1016/j.alcohol.2014.03.001
Johnson, A. C., & Greenwood-Van Meerveld, B. (2016). The Pharmacology of Visceral Pain. Advances in pharmacology (San Diego, Calif.), 75, 273–301. https://doi.org/10.1016/bs.apha.2015.11.002
Kandel, E. R., Dudai, Y., & Mayford, M. R. (2014). The Molecular and Systems Biology of Memory. Cell, 157(1), 163–186. https://doi.org/10.1016/j.cell.2014.03.001
Lee, G. J., Park, J. H., & Park, H. K. (2008). Microdialysis Applications in Neuroscience. Neurological research, 30(7), 661–668. https://doi.org/10.1179/174313208X289570
Leroy, A., Foucher, J. R., Pins, D., Delmaire, C., Thomas, P., Roser, M. M., Lefebvre, S., Amad, A., Fovet, T., Jaafari, N., & Jardri, R. (2017). fMRI Capture of Auditory Hallucinations: Validation of the Two-Steps Method. Human brain mapping, 38(10), 4966–4979. https://doi.org/10.1002/hbm.23707
Liu, Z., Ding, L., & He, B. (2006). Integration of EEG/MEG with MRI and fMRI. IEEE engineering in medicine and biology magazine : the quarterly magazine of the Engineering in Medicine & Biology Society, 25(4), 46–53. https://doi.org/10.1109/memb.2006.1657787
Lodish H., Berk A., Zipurksy S. L. et. al., editors. (2000). Molecular Cell Biology, 4th edition. Freeman, p 94, 140, 147-148, 268-269.
Malik, A. N., Vierbuchen, T., Hemberg, M., Rubin, A. A., Ling, E., Couch, C. H., Stroud, H., Spiegel, I., Farh, K. K., Harmin, D. A., & Greenberg, M. E. (2014). Genome-wide Identification and Characterization of Functional Neuronal Activity-Dependent Enhancers. Nature neuroscience, 17(10), 1330–1339. https://doi.org/10.1038/nn.3808
Mayford, M., Siegelbaum, S. A., & Kandel, E. R. (2012). Synapses and Memory Storage. Cold Spring Harbor perspectives in biology, 4(6), a005751. https://doi.org/10.1101/cshperspect.a005751
Maze, I., Shen, L., Zhang, B., Garcia, B. A., Shao, N., Mitchell, A., Sun, H., Akbarian, S., Allis, C. D., & Nestler, E. J. (2014). Analytical Tools and Current Challenges in the Modern Era of Neuroepigenomics. Nature neuroscience, 17(11), 1476–1490. https://doi.org/10.1038/nn.3816
National Human Genome Research Institute. (July 2017). An Overview of the Human Genome Project. Accessed July 17, 2017 at https://www.genome.gov/12011238/an-overview-of-the-human-genome-project/
National Institute of Mental Health. (2017). Brain Stimulation Therapies. Accessed July 17, 2017 at https://www.nimh.nih.gov/health/topics/brain-stimulation-therapies/brain-stimulation-therapies.shtml
Olgiati, S., Quadri, M., & Bonifati, V. (2016). Genetics of Movement Disorders in the Next-Generation Sequencing Era. Movement disorders, 31(4), 458–470. https://doi.org/10.1002/mds.26521
Perry, R. H., Blessed, G., Perry, E. K., & Tomlinson, B. E. (1980). Histochemical Observations on Cholinesterase Activities in the Brains of Elderly Normal and Demented (Alzheimer-type) Patients. Age and ageing, 9(1), 9–16. https://doi.org/10.1093/ageing/9.1.9
Purves D, Augustine GJ, Fitzpatrick D, et al., editors. (2008). Neuroscience. 4th edition. Sinauer Associates, Inc. p 3-5, 16-17, 19-21, 25-27, 181-187, 465, 559, 673-674, 715-717.
Sejnowski, T. J., Koch, C., & Churchland, P. S. (1988). Computational Neuroscience. Science (New York, N.Y.), 241(4871), 1299–1306. https://doi.org/10.1126/science.3045969
Sokolowski M. B. (2001). Drosophila: Genetics Meets Behaviour. Nature reviews. Genetics, 2(11), 879–890. https://doi.org/10.1038/35098592
Svoboda, K., & Yasuda, R. (2006). Principles of Two-Photon Excitation Microscopy and its Applications to Neuroscience. Neuron, 50(6), 823–839. https://doi.org/10.1016/j.neuron.2006.05.019
Turek, F. W., Pinto, L. H., Vitaterna, M. H., Penev, P. D., Zee, P. C., & Takahashi, J. S. (1995). Pharmacological and Genetic Approaches for the Study of Circadian Rhythms in Mammals. Frontiers in neuroendocrinology, 16(3), 191–223. https://doi.org/10.1006/frne.1995.1007
US National Library of Medicine, National Institutes of Health. (2017). Genetics Home Reference – Huntington Disease. Accessed July 17, 2017 at https://ghr.nlm.nih.gov/condition/huntington-disease#genes
Usdin, K., & Kumari, D. (2015). Repeat-mediated Epigenetic Dysregulation of the FMR1 Gene in the Fragile X-related Disorders. Frontiers in genetics, 6, 192. https://doi.org/10.3389/fgene.2015.00192
Yoshino, K., Oka, N., Yamamoto, K., Takahashi, H., & Kato, T. (2013). Functional Brain Imaging Using Near-infrared Spectroscopy During Actual Driving on an Expressway. Frontiers in human neuroscience, 7, 882. https://doi.org/10.3389/fnhum.2013.00882