How Inhibitory Neurons Shape the Brain’s Code
- Published6 Oct 2021
- Author Alexis Wnuk
- Source BrainFacts/SfN
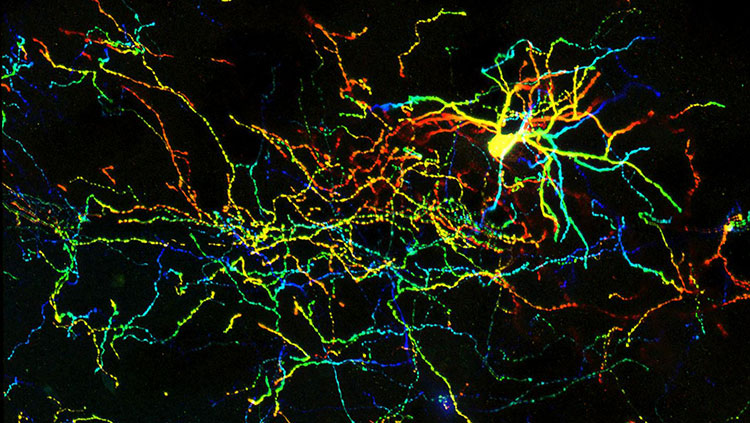
As a kid, Péter Somogyi helped the local forestry company study the breeding behavior of the forest’s birds, scaling the trees to count the number of eggs in the nest boxes he and his friends had rigged up. These days, the Oxford scientist studies the human brain. His lab works with tissue samples donated by surgery patients. After slicing the tissue into thin sheets, they bathe it in a saline and oxygen solution so they can record the activity of the cells. “A slice that we take out at 10 a.m. is still producing activity at 10 a.m. the next day,” he says. “Twenty-four hours later, this piece of human brain is still working furiously in the dish.”
Somogyi wants to understand how the cells are talking to each other. Early in his career, he was inspired by the renowned Hungarian neuroscientist János Szentágothai. “He realized early on in the 1930’s that to explain the brain, to explain our behavior, to explain our thought processes, you needed a definition of the neurons and how they are connected,” Somogyi says. “This seems trivial now — how could you explain it otherwise? But at the time, it was not.”
This philosophy guides Somogyi and fellow neuroscientists György Buzsáki and Tamás Freund. For cataloging and mapping of the brain’s inhibitory circuits, the trio earned the inaugural Brain Prize in 2011. We spoke with them about their work and its importance for human health.
What are inhibitory neurons?
The bulk of the brain’s neurons are excitatory — when they fire, they activate other neurons and propagate electrical signals throughout the brain. Inhibitory neurons do the opposite. The volley of chemical messages restrain, or inhibit, other neurons, making them less likely to fire messages of their own.
There are all kinds of different inhibitory neurons, many with colorful names to describe their unique shapes: the branched nerve fibers of basket cells appear to encase their targets in a mesh, while the axons of chandelier cells resemble candlesticks on an ornate light fixture.
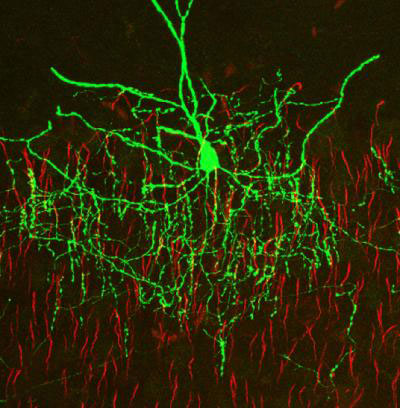
It was in the chandelier cells that Somogyi made his first significant discovery. As a junior scientist at Semmelweis University, Somogyi studied the cerebellum. But his real interest was the cerebral cortex. So, he started working on it in secret, coming into the lab on weekends or staying into the evening. Szentágothai, who first described the chandelier cells, believed their candlestick-like axons wrapped about the dendrites of pyramidal neurons. Somogyi set out to prove his hypothesis. But when he peered into the microscope, he saw that the axons wrapped around a different part of the neuron: the axon initial segment, the node where electrical signals are generated. “I cannot describe … this eureka moment,” Somogyi says. “The moment of insight when you see something and you know, ‘that’s important.’” Because the electrical signals propagating down the axon are generated at the axon initial segment, a neuron that exclusively targets this region must be important. He rushed to Szentágothai to tell him that his theory was wrong. Unconvinced, Szentágothai insisted he was looking at an entirely different breed of neuron because he was looking at the rat brain — Szentágothai identified chandelier cells in monkeys. So, Somogyi named them axo-axonic cells. “Gradually, he accepted that it’s the same cell,” Somogyi says. “But that’s how the name ‘axo-axonic cell’ was born.”
Why is inhibition important?
Inhibitory neurons comprise just 10-20% of all the neurons in the brain, but they play an outsized role in packaging and transmitting information in the brain.
Buzsáki, a professor at New York University’s Langone Medical Center, likens this role to the natural breaks in language. “The reason I can communicate with you is because I pause between the words and I pause between sentences,” he says. “In other words, we segment the information we send. Now in the brain, the natural way how information can be segmented is inhibition.”
Each of the different kinds of inhibitory neurons is like a different kind of punctuation. “This one is a comma. This other one is a full stop, and so on.”
Inhibition also allows neurons to synchronize their firing, giving rise to rhythmic oscillations of activity, or brain waves. These are powerful tools for transmitting complex information cheaply. Buzsáki illustrates this with another analogy: imagine going to a theater performance in Europe. When people like the show, they clap their hands rhythmically. “When you clap your hands rhythmically with others, then the surge of noise is much more powerful … but it costs the same amount of muscle power” as clapping out of sync.
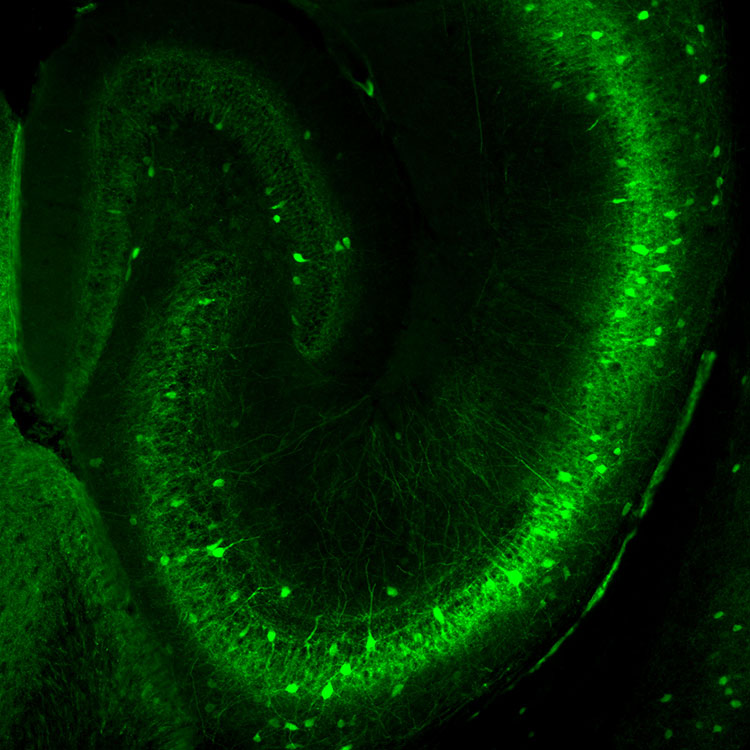
Sometimes, inhibition actually excites cells down the line. In a 1988 study, Freund discovered a group of inhibitory neurons arising from an area of the brain called the septum. The neurons reach into the hippocampus where they interact exclusively with other inhibitory neurons charged with tamping down the activity of excitatory cells. By impeding these inhibitors, the cells essentially release the brakes on the excitatory neurons. That turns out to be important. The septal pathway carries information about motivation and emotions — our “internal world,” as Freund and Buzsáki put it. By feeding information about our internal world to the brain’s memory center, these neurons may improve the storage and recall of the memories we deem most important. This may explain why “we perfectly remember events, regardless of how long ago they happened, if they have intense emotional attributes,” Freund, now the President of the Hungarian Academy of Sciences, said in an email.
What role does inhibition play in neurological and psychiatric disorders?
Inhibitory neurons shape the brain’s electrical syntax. When they don’t work properly, things go awry. “In every single psychiatric disease, there is some kind of an oscillation problem,” Buzsáki says. Scientists have zeroed in on specific inhibitory neurons involved in some of these diseases. For instance, one of the key pathological changes in schizophrenia is the functional loss of inhibitory neurons expressing a protein called parvalbumin. These neurons — which include chandelier cells — “are the key players for rhythms” and they don’t fire as much as they should in people with schizophrenia, Buzsáki says.
Most inhibitory neurons communicate using the neurotransmitter GABA, or gamma-aminobutyric acid. GABA binds to synaptic receptors to dampen nerve cell activity. It also binds to a separate group of receptors sprinkled all over a neuron’s surface. These receptors act like GABA sensors, monitoring the concentration of GABA in the extracellular space. If GABA levels go up, these receptors open up and let negatively charged chloride ions flood into the cell. This flux determines how excitable or sluggish the target cell is.
Recently, scientists learned the GABA sensors were also involved in postpartum depression. Hormone surges during pregnancy increase this tonic current. To compensate, brain cells respond by yanking GABA sensors from their membranes. When hormone levels return to normal after the child is born, the current also returns to normal but there are no longer enough GABA sensors. “That’s the underlying condition for postpartum depression,” Somogyi says. In 2019, the U.S. Food and Drug Administration approved the first treatment to address this. “This is clearly a very important step forward,” Somogyi says.
Freund’s research interests have shifted somewhat to endocannabinoid signaling. Endocannabinoids are natural substances produced by the body with similar actions to the chemicals in marijuana. He discovered that they bind to receptors on the nerve terminals of both inhibitory and excitatory neurons. When they bind, they restrain the release of neurotransmitters. In effect, the endocannabinoid receptors are neural circuit breakers. The research “led to the discovery of a molecular machinery that controls the homeostasis of both excitatory and inhibitory transmission, and its malfunction can lead to anxiety or epilepsy,” Freund says. Scientists are exploring this molecular machinery to identify new treatments for these disorders.
CONTENT PROVIDED BY
BrainFacts/SfN
References
Freund, T. F. (2003). Interneuron Diversity series: Rhythm and mood in perisomatic inhibition. Trends in Neurosciences, 26(9), 489–495. https://doi.org/10.1016/S0166-2236(03)00227-3
Freund, T. F., & Antal, M. (1988). GABA-containing neurons in the septum control inhibitory interneurons in the hippocampus. Nature, 336(6195), 170–173. https://doi.org/10.1038/336170a0
Howard, A., Tamas, G., & Soltesz, I. (2005). Lighting the chandelier: New vistas for axo-axonic cells. Trends in Neurosciences, 28(6), 310–316. https://doi.org/10.1016/j.tins.2005.04.004
Katona, I., & Freund, T. F. (2008). Endocannabinoid signaling as a synaptic circuit breaker in neurological disease. Nature Medicine, 14(9), 923–930. https://doi.org/10.1038/nm.f.1869
Soltesz, I. (2011). The Brain Prize 2011. Trends in Neurosciences, 34(10), 501–503. https://doi.org/10.1016/j.tins.2011.08.006
Swanson, O. K., & Maffei, A. (2019). From Hiring to Firing: Activation of Inhibitory Neurons and Their Recruitment in Behavior. Frontiers in Molecular Neuroscience, 12, 168. https://doi.org/10.3389/fnmol.2019.00168
What to Read Next
Also In Cells & Circuits
Trending
Popular articles on BrainFacts.org